2024-2025 Soft Matter Graduate Fellowship Recipients
Posted in News Story
The ISM is excited to introduce the 2024-2025 Soft Matter Graduate Fellowship recipients: Micaila Curtis, Shae London, and Wayan Fontaine-Seiler!
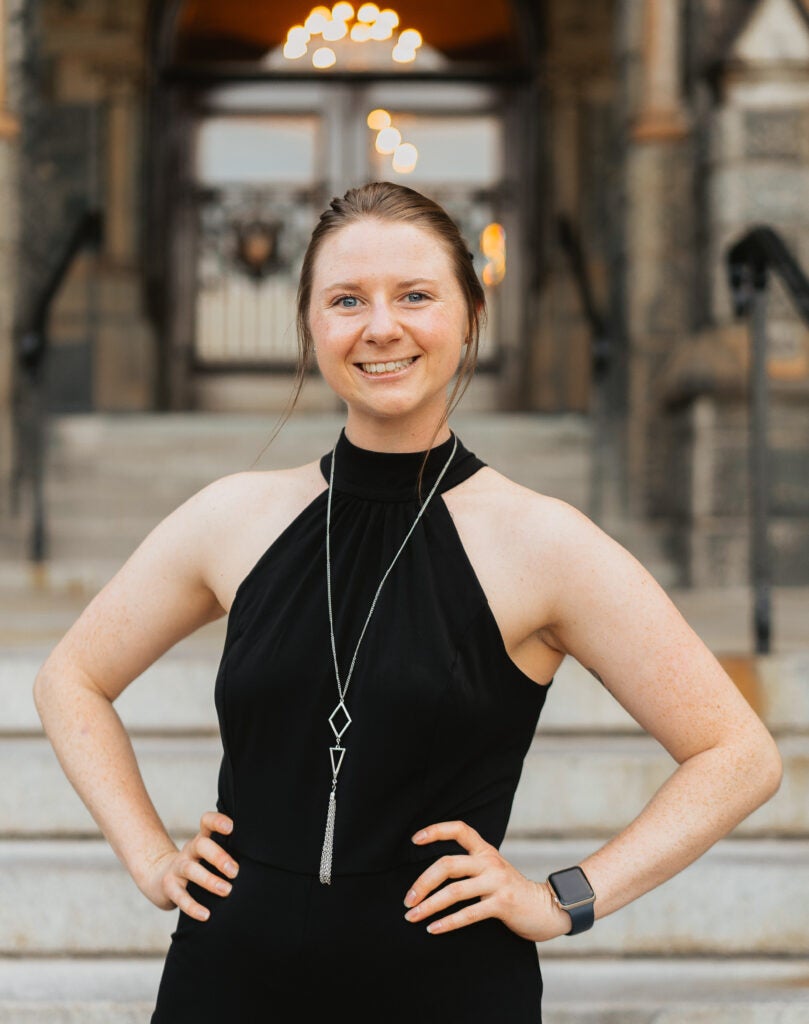
Micaila Curtis
Advisor: Professor Stella Alimperti
My research sits in the intersection between biochemistry and mechanical property characterization for bone tissue regeneration. The aging and active populations are increasingly burdened by musculoskeletal degenerative diseases and limited or challenging clinical interventions for bone diseases and fractures. Therefore, we are developing new bioengineered tools for recapitulating precision-medicine-grade human disease models in vitro to identify molecular targets for therapeutic interventions, as well as generating 3D-bioprinted implantable scaffolds for bone tissue regeneration in vivo. Measuring the mechanical properties of soft materials includes the use of rheology, AFM, DMA, and SEM to support our chemical analysis from techniques such as FTIR, EDS, XRD, and NMR, which is then paired with data from molecular and cellular assays for a comprehensive analysis of the generated extracellular matrix microenvironment. The use of equipment and top-tier training by faculty at the ISMSM have greatly supported this project so far and will support continued analysis of 3D-bioprinted materials for optimal biocompatibility and stimulation of tissue regeneration from generated biomaterials. We have already seen that the characterization of generated and native bone tissue mechanical properties is pertinent to the applicability of our in vitro microfluidics models to biomedical innovation, but it also supports the generation of 3D bioprinted scaffolds for implantation in hopes to support bone reconstruction and highlight the host integration of implantable bone through surgical grafting efforts.
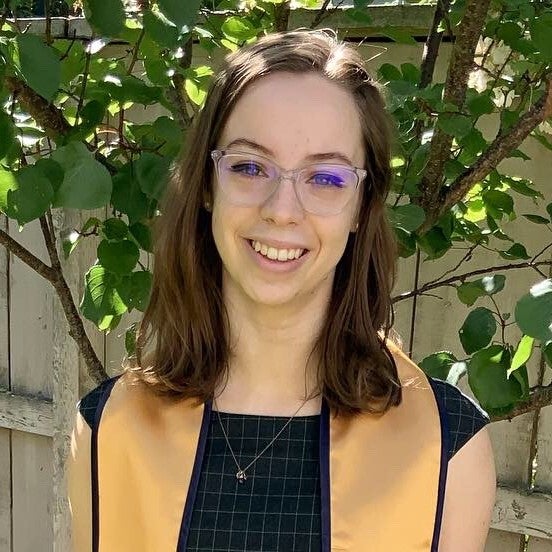
Shae London
Advisor: Professor Jennifer Swift
Since joining the Swift group in Aug 2023, I have initiated several follow-up studies on synthetic ammonium urate to (1) assess the limits of ammonia capture and (2) elucidate the role of K+ in biogenic samples.
Ammonium urate was previously synthesized in our lab by reacting UA with ammonium hydroxide (NH4OH) in a 1:800 ratio, yielding a mixture of ammonium urate and ammonium urate hydrate (AUH). To more closely simulate biogenic conditions, the solution ammonia concentration was serially diluted from 800:1 to 1:1 NH4OH:UA. Once a molar ratio of 5:1 NH4OH:UA was reached, a jelly-like suspension formed over 3 days. The 5:1 and 2:1 NH4OH:UA samples had PXRD patterns comparable to AUH and similar desolvation temperatures by differential scanning calorimetry. Ongoing efforts to characterize the jelly-like material with SEM and other methods are underway, and preliminary analyses suggest the formation of a weak fibrillar network.
Interest in K+ stems from results of a dietary study in which rattlesnakes fed either rodents or hornworms were found to produce “urates” with very different potassium levels. K+ levels in the “urates” of hornworm-fed snakes were high (up to 15 wt %) and also showed evidence of potassium urate monohydrate. The structural models for the AUH in these “urates” also exhibit some unique features in the PXRD patterns which have been the subject of much debate among collaborators. The substitution of K+ in some NH4+ sites in the AUH lattice may explain these effects. To test this hypothesis, I have prepared AUH from solutions with varying UA:NH4OH:KCl ratios. I have explored the conditions that yield the jelly-like phase, 5:1 NH4OH:UA, and varying the molar ratio of KCl from 1 to 0.25. In this series of reactions, the size of the settled layer seems to correlate with the amount of KCl in the reaction solution. Interestingly, the larger layers seem to more readily collapse upon agitation while the smaller layers hold their structure until filtration and washing. Presently, the differences in the networks are currently being investigated with SEM. The PXRD patterns of these samples are also interesting since the relative intensities differs from other AUH samples. Understanding the nature of these changes will be a focus of future work.
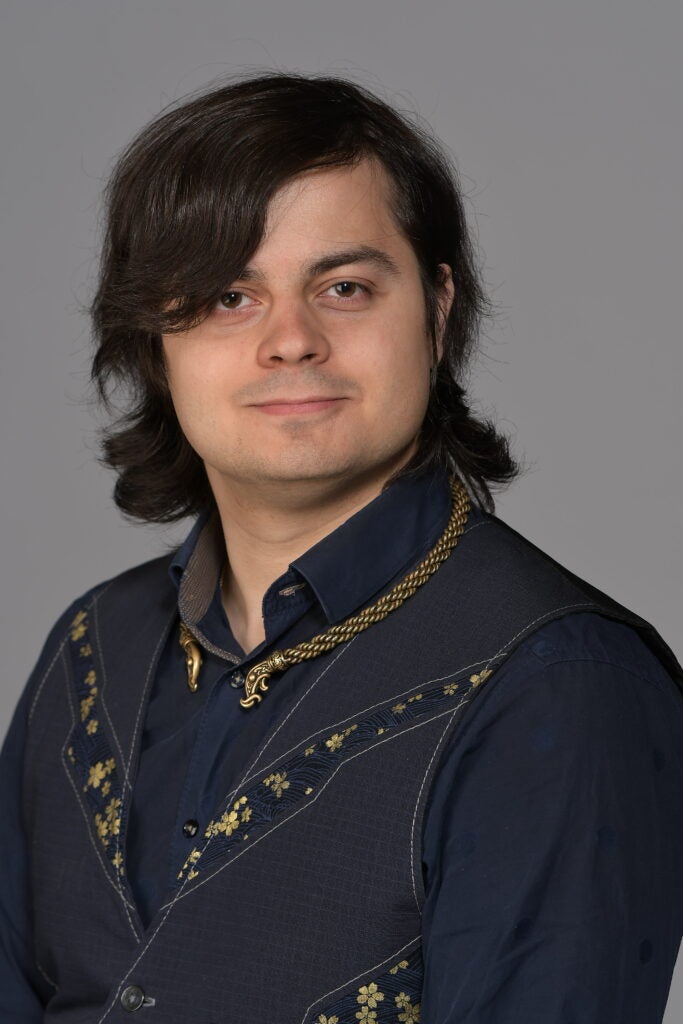
Wayan Fontaine-Seiler
Advisor: Professor Daniel Blair
My research aims to expand the tools that can be used to characterize and quantify the mechanical properties of materials under large strains. As complex composite bio-derived and bio-compatible networks and polymers are becoming of increasing importance in biomedical research, the need to fully understand their mechanical properties, their origins and the potential parameters controlling these properties, is paramount for biomaterial engineering. From the three dimensional culture of cells and cell structures, the advent of phantoms and scaffolds for the artificial growth of organs and 3D bioprinting, the understanding of their mechanical properties, and the microscopic mechanisms they originate from, is crucial for the future of biomedical research and bio-engineering.
During manufacturing and production stages as well as their use, such materials can undergo large deformations beyond their linear regime and can display complex nonlinear mechanical behaviors as well as undergo substantial modifications of their microstructure that can affect their properties and their performance in the applications for which they are designed. Unfortunately, standard experimental analysis frameworks and methods capable of analyzing such mechanical behaviors and approachable enough are lacking. This is due, in part, to the wide variety and complexity of mechanical responses these materials display as well as the competition between different analytical approaches and their complexity. Consequently, the standard toolbox of the biomaterial engineer often lacks a required comprehensive framework to describe and quantify these complex behaviors. To answer this need, our work focuses on the development of experimental analysis tools to characterize the complex mechanical responses under dynamic large strain deformations and provide simple, intuitive and experimentally driven parameters to quantify different aspects of the complex response. More precisely, we are developing a statistical and geometrical analysis extension over a time-resolved analysis framework for Large Amplitude Oscillatory Strain (LAOS) rheology to extract physically relevant variables and useful parameters that can constitute a “first order” approximation of complex rheological behaviors.
Through this extension to the framework, we hope to provide, not only a useful tool for the engineering of novel functional biomaterials, but also participate in the foundation for a possible fingerprinting method for the nonlinear responses of complex materials. We also believe our tool can form part of the toolbox for the fundamental understanding of the microscale mechanics leading to the observed bulk properties of such materials through experimental and computational means.